Update: This post is now outdated! We ended up using a similar, but much improved, spectral interference method using a broadband laser source instead of the continuous-wave HeNe laser. The method is described in the publication on the spectrometer design.
Here is a quick rundown on how the delay stages are calibrated to relate the rotation angle to the delay time of the laser pulse.
An interferometer is built by inserting a mirror/beamsplitter combination after the delays to interfere pairs of the four beams. A Helium-Neon (HeNe) laser is used as the light source, as the atomic emission at 632.8 nm is very well defined — a requirement to make a nice interference pattern and for use in the calibration fit equation.
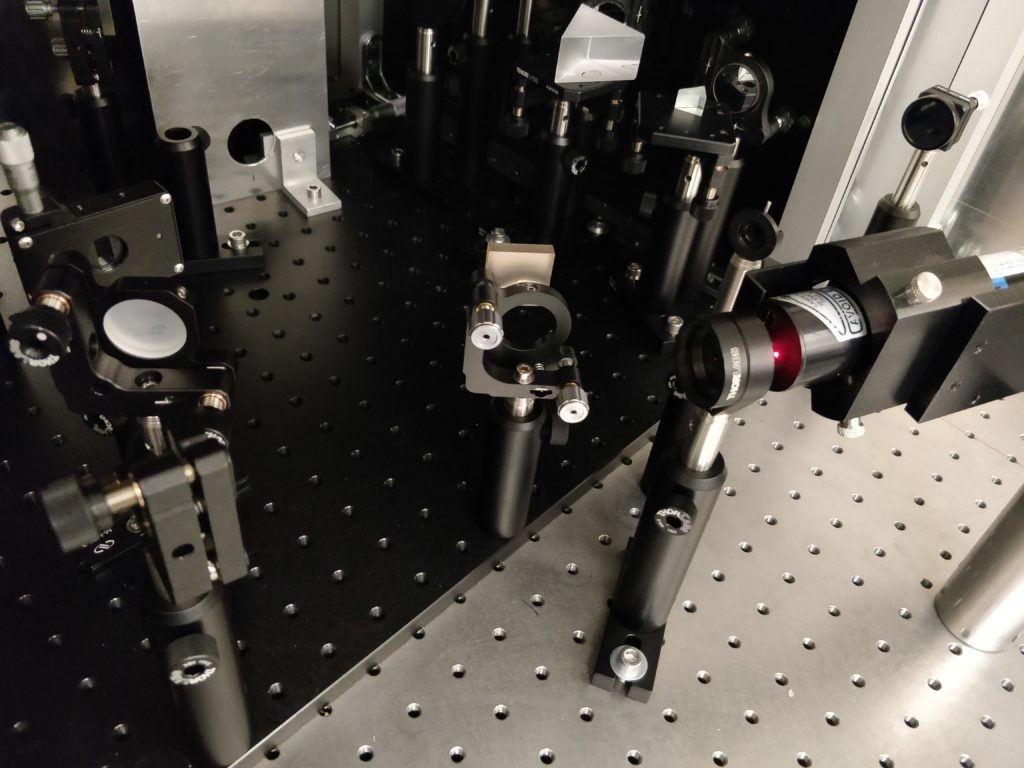
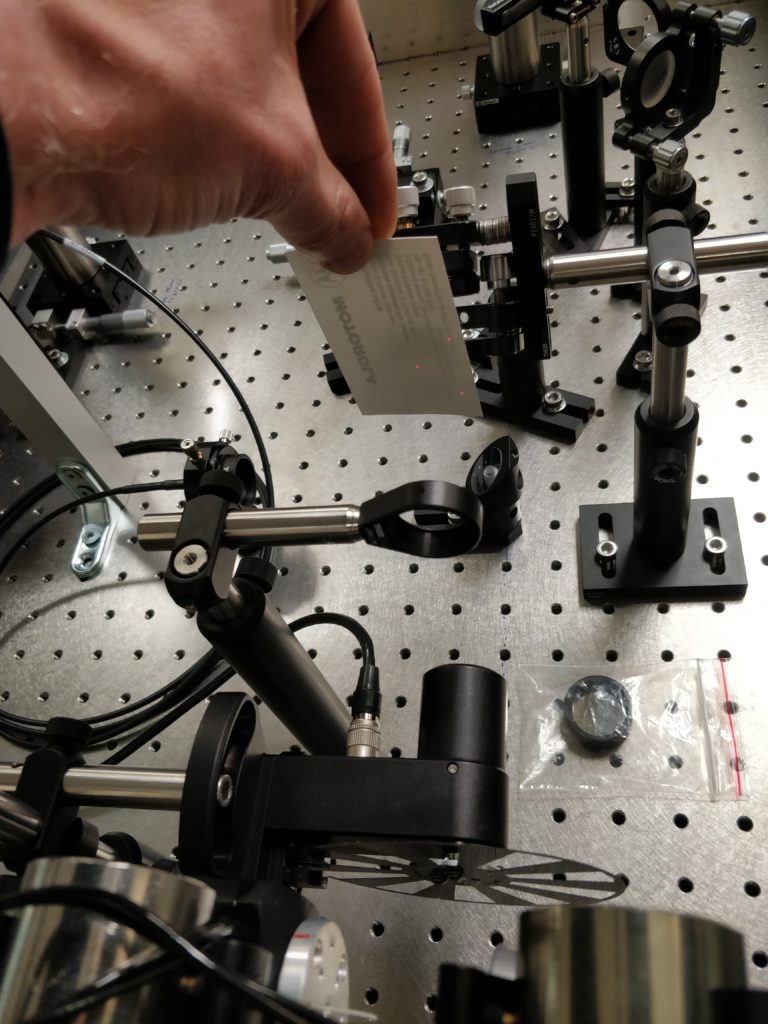
Here we show the vertical configuration of the beamsplitter/mirror combination, which interferes the top and bottom beams, directing them downwards towards the table. The beam is directed to a fibre-optic which feeds the spectrograph and camera.
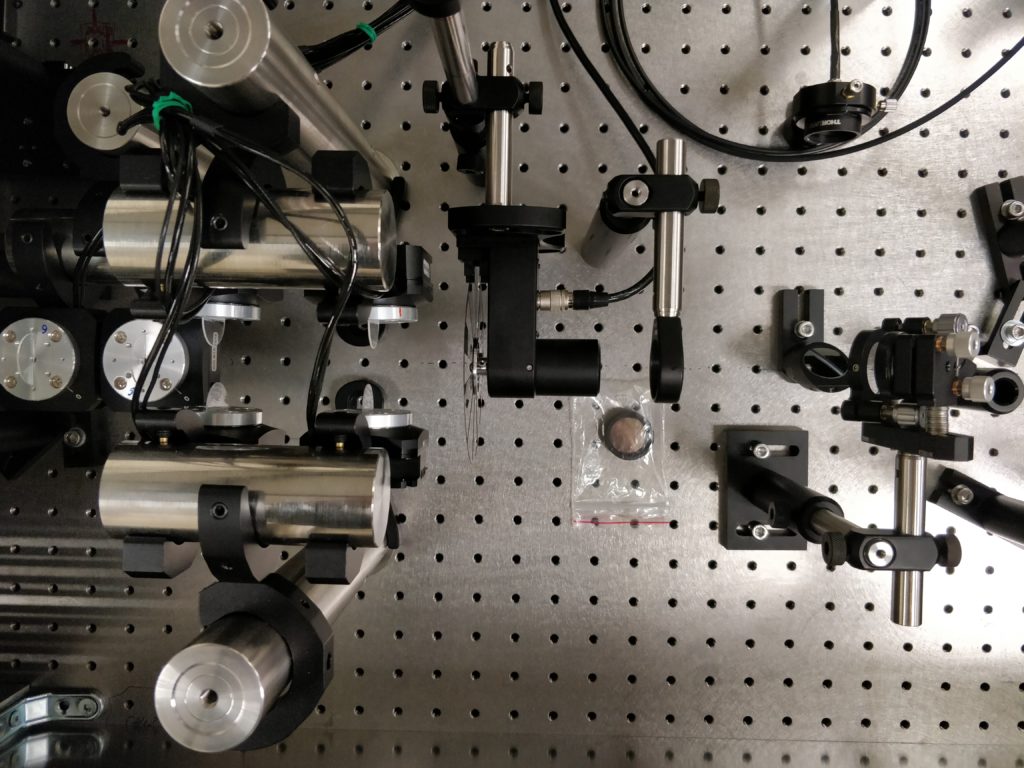
I think this is the first look here at some of the spectrometer software! The intensity at the 632.8 nm laser line is collected as a function of one of the rotational stage angles. The rotational stage is swept between 0° (glass perpendicular to beam) to around 45°. An interference pattern is generated.
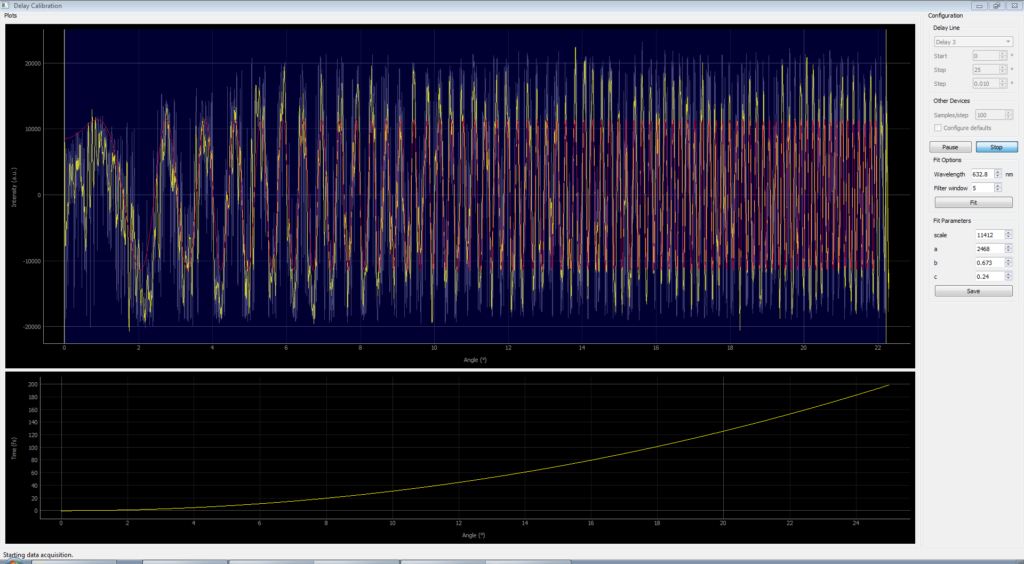
The grey trace is the raw intensity data as a function of rotational stage angle. The yellow trace is with a Savitzky–Golay noise filter applied. The red trace, and the yellow curve in the bottom panel show the theoretical angle-to-delay relationship. As the data is collected, or when the “Fit” button pressed, the fit is performed and the three fitting parameters (α, β, γ) are determined. The fit can also be adjusted in realtime as the parameters are changed manually.
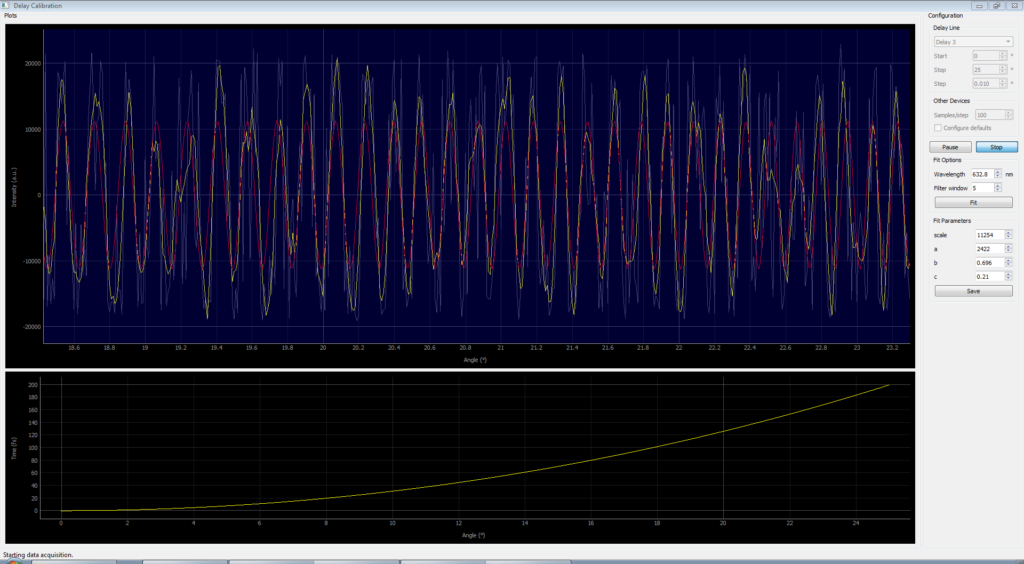
The theoretical model of the glass angle vs delay match the data very well across the entire range of rotation angles. That’s good, as it’s pretty fundamental to the whole spectrometer design!